CMEDE Research
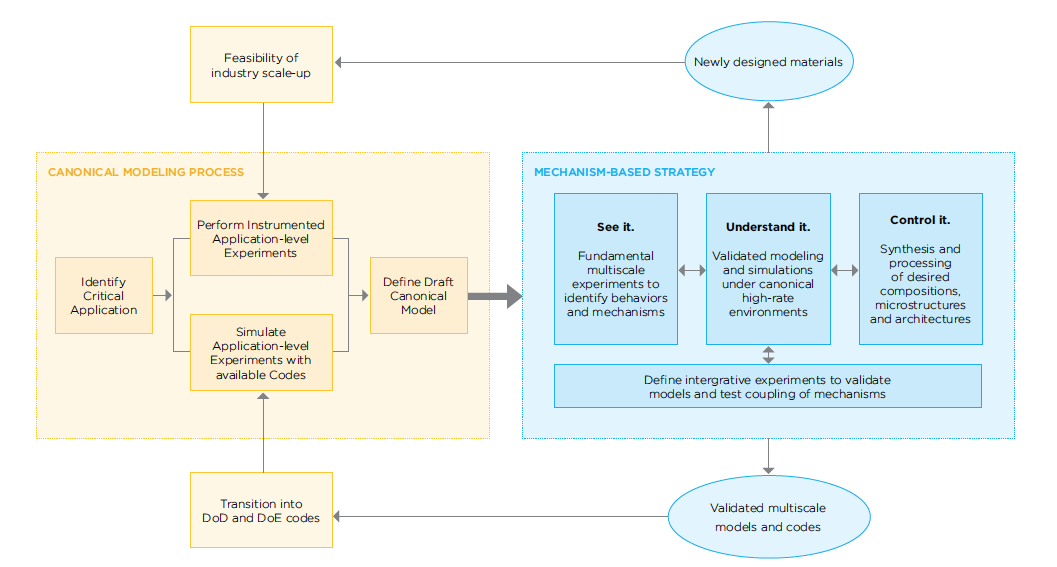
Overall design strategy for protection materials. Left-hand boxes are driven by ARL, while right-hand boxes are driven by the MEDE Consortium.
Research Strategy
The objective of the MEDE program was to develop the technical and workforce capability to design, create, and optimize novel material systems that exhibit revolutionary performance in extreme dynamic environments. Achieving this objective requires a new paradigm for materials research and workforce development. One cannot use the classical materials science structure-properties-performance approach because path-dependent and time-dependent failure processes are involved in these dynamic environments, and optimal solutions may not exist in the traditional design space. Instead, we must design with knowledge of the dynamic failure processes (mechanisms) that are involved in the actual application.
To achieve the MEDE program objectives, research activities were focused on a materials-by-design process involving a canonical model and a mechanism-based strategy as shown in the below figure. We established a canonical model for each model material under investigation. A canonical model is defined as: “A simplified description of the system or process, accepted as being accurate and authoritative, and developed to assist calculations and predictions.” Typically such a canonical model defines key variables and their ranges, defines critical mechanisms, and then prioritizes the variables and mechanisms. Beginning with a canonical model allows a large group of researchers to ensure that efforts are relevant in terms of both science and application.
Once the canonical description is established, researchers can then proceed with the mechanism-based strategy. Researchers seek to see the mechanisms during the extreme dynamic event, to understand them through multiscale models, and to control them through synthesis and processing. Understanding the mechanisms through multiscale models provides the capability to define integrative experiments and to test the coupling of mechanisms. This information leads to validated models and codes, which feed back into the canonical model, by transitioning into Department of Defense (DoD) and Department of Energy (DoE) codes. Similarly, controlling the mechanism through synthesis and processing leads to newly designed materials for the canonical environment. Industry helps to determine the scale-up feasibility of these newly designed materials, which are then fed back to the experiments in the canonical modeling effort.
Materials by Design Process
Throughout the program, each CMRG has progressed through discovery, integration and transition for multiple candidate materials. The See/Understand/Control mechanism-based framework has been a constant throughout the program. The final and critically important piece of the program is workforce development, which encompasses all aspects of MEDE. Mechanism-based, See It-Understand It-Control It Paradigm.
• See It: Observe mechanisms through testing in extreme environments
• Understand It: Computational models to understand mechanisms
• Control It: Synthesis & processing to control mechanisms
• Discovery Phase: evaluation and control of key mechanisms
• Integrative Phase: consider competition between key mechanisms in canonical model
• Transition Phase: codes, datasets, scaled-up material specimens transitioned to DEVCOM ARL
• Workforce Development: throughout and across the entire program.
Research Activities
The MEDE program examined one model material in each of the following materials classes: ceramics, composites, metals, and polymers. The discoveries and insights developed can be used for other materials in the same class. Collaborative research within each material system was planned, executed and overseen by a Collaborative Materials Research Group or CMRG. Thus, there are three CMRGs: the Ceramics CMRG, the Composites CMRG, and the Metals CMRG. The role of the CMRG is to coordinate and oversee all research within the Consortium on that material system, to ensure that there is appropriate communication between experimental, modeling and processing activities. Additionally, there are integrative research activities which cross-cut multiple CMRGs.
Ceramics: Boron Carbide
Boron carbide is the model material for the Ceramics CMRG because it has the unrealized potential of dramatic improvements in ballistic performance for soldier and vehicular protection at very low weight. The Ceramics CMRG seeks to understand and control the dynamic failure processes in this protective ceramic material and to improve its dynamic performance by controlling mechanisms at the atomic and microstructural levels through multiscale modeling, advanced powder synthesis, control of polytypes, and microstructural improvements.
Application: Boron carbide is one of the component materials used to protect soldiers and military vehicles from blast and ballistic threats.
Composites: S-2 Glass/Epoxy
Composite materials subjected to dynamic loads are essential examples of high performance systems in the conventional sense. In order to focus on the complexities raised by the interfaces and architectures, S-2 Glass/Epoxy is the model system for the Composites CMRG. The Composites CMRG develops the fundamental understanding of the role of interfaces, component interactions, and composite architecture over the full range of length scales and time scales that are manifested in the system during the dynamic event.
Application: S-2 Glass/Epoxy provides a strong, structural backing system to support protective plates for military vehicles.
Metals: Magnesium
The magnesium alloy system is the model material for the Metals CMRG because it is the lightest-weight structural metal that offers the potential of approaching steel-like ballistic performance while using conventional low-cost and time-tested processing techniques. We are enhancing the dynamic performance of this hexag-onally-close-packed metal using experimentally validated modeling and alloy design to control dynamic strengthening and failure mechanisms, including deformation twinning.
Application: In comparison to steel, magnesium offers the potential for a lightweight metal system that could enhance the deployability and protection of military vehicles.