The following article was originally published on 11/15/23 by Lawrence Livermore National Laboratory (LLNL) and features Sophie Parsons, Harry Radousky, and other MSEE collaborators in Research Area 4 – Focus Area 2: Direct Laser Impulse.
Article by Anne M. Stark
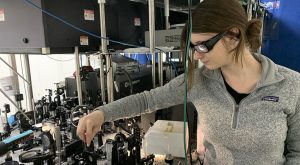
Ph.D. student Sophie Parsons conducts laser alignment work on an ultrafast pulse table-top laser system modified for sub-nanosecond shock compression and velocimetry. Photo by Alison Ackerman/UCSD.
Understanding laser material interactions has applications that include inertial confinement fusion, material research and equation of state studies.
Laser ablation, and specifically increasing the pressure that can be achieved from a laser system, is a longstanding topic of scientific research with implications ranging from damage in layered devices like solar cells and electronic devices to improving hydrodynamic code simulations.
The physics governing a laser interacting with a material are complex and dependent on the intensity and time scale of the incident laser pulse. In new research appearing in Applied Physics Letters, a team of researchers from Lawrence Livermore National Laboratory (LLNL) and University of California, San Diego (UCSD) conducted experiments to see how aluminum reacts to a laser under extreme pressure when a tamper material is used on picosecond (ps) time scales.
One method increases the pressure generated from the laser by using a tamper material (a material that sits between the sample material and the laser). When the drive laser is turned on to transparent materials, they confine the plasma generated by the laser, mitigating the effects of plasma blowoff. Confining the volume increases the energy remaining at the interface, thus increasing the resulting pressure. Tampers have been shown to increase the pressure in the material by four to 50 times as compared to an experiment where the laser is shined directly on the ablator.
“Attempts to model the details of this set of experimental data with standard, available radiation-coupled hydrodynamic codes makes clear that more physics is needed in these simulations to accurately predict the impact of the tamper material on the pressure generation and the depth of non-solid aluminum,” said lead author Sophie Parsons, a UCSD graduate student involved in LLNL’s Academic Cooperation Program.
The result: shock velocities observed on these 100-picosecond timescales do not match hydrodynamic simulations. The team’s experimental data can be used as a benchmark against simulations to better understand the laser-matter interactions governing the shock formation.
The experiment involved a 100-picosecond laser used to probe the pressure generation, depth of the non-solid ablator and the non-linear optical effects through tamper materials. Samples consisted of an aluminum ablator with tampers of sapphire and coverslip glass, respectively. In general, the sapphire tamped sample achieves higher pressures at lower laser intensities as compared to the coverslip glass tamped sample.
“We observed higher pressures at lower laser intensities in the sapphire tamped sample compared to coverslip glass which is expected given the material properties of these two tampers,” said LLNL physicist Mike Armstrong. “We also found that traditional hydrodynamic codes do not accurately predict these pressures for the 100-picosecond time scale, nor do they predict the amount of non-solid aluminum observed in experiments at these short times.”
The project is part of the Materials Science in Extreme Environments University Research Alliance (MSEE URA). “The MSEE collaboration is a great opportunity to involve graduate students in research topics of importance to the Lab,” said physicist Harry Radousky, who is the LLNL PI for the MSEE project. (A video describing this experiment can be found on LLNL’s YouTube page..
Other researchers include Ross Turner, a UCSD graduate student involved in LLNL’s Academic Cooperation Program as well as Javier Garay and Farhat Beg of UCSD. This work was funded by the Defense Threat Reduction Agency.